1. Introduction
Polylactic acid (PLA) is a distinct catalyst for neocollagenesis.[1] PLA has been widely used since its approval by the U.S. Food and Drug Administration as an injectable agent for augmentation of facial soft tissues in treating lipoatrophy, owing to its significant therapeutic efficacy.[2] The demand for new biomaterials, which would provide a good clinical outcome where tissue volume restoration is required, is expected to increase even further.[2] In this regard, researchers worldwide are making efforts to develop new, even more effective PLA-based dermal fillers. One such new-generation medicinal product is discussed below.
Chronological aging of the skin is a complex process controlled by numerous internal and external factors,[3] one of the most important of which is the gradual degradation of the dermis network of collagen fibers. The extracellular matrix (ECM) mainly consists of collagen fibers of types I and III, which form a framework wherein fibroblasts producing the matrix are fixed by transmembrane binding to the integrin receptor.[4] This stretches fibroblasts, ensuring cell integrity and balanced production of collagen and matrix-destroying metalloproteinases (MMPs).[5] In addition to MMPs, fibroblasts can also release tissue inhibitors of MMPs.[6] In aging skin, collagen fibers fragment, and fibroblasts lose their stability and are destroyed.[7] In the damaged matrix of the dermis, fibroblasts make contact only with a small number of collagen fibers or collagen fragments, leading to changes in their spatial structure.[7] In the absence of tension and stretching, fibroblasts stop synthesizing collagen and switch to producing MMPs, resulting in even greater matrix degradation.[7] A vicious circle is formed wherein the triggered internal mechanisms of skin aging are promoted by unfavorable external factors. The decrease in collagen production and increase in MMP production can lead to skin laxity.[8] Therefore, the primary cause of skin aging manifestations involves a progressive decrease in the content of principal structural elements of the ECM (collagen, elastin, and glycosaminoglycans) and a change in their characteristics.[8] Despite differences in lifestyle and environment, the first signs of aging of a person’s face appear at the age of 20–30 years.[9] The network architecture of the dermis plays a leading role in determining the mechanical behavior of the skin as a whole. Skin with highly dense load-bearing ECM (mostly collagen) shows the greatest resistance to stretching and ptosis (Fig. 1).[10] With the onset of menopause, the collagen content of the skin decreases steadily every year. In the first 5 years, up to 30% of skin collagen is lost,[11] causing loss of skin elasticity and firmness, decrease in its thickness, and formation of wrinkles.
2. Importance and activation method of collagen
Since collagen loss is the most significant factor in the emergence of external signs of skin aging, most cosmetological antiaging methods precisely aim to activate the synthesis and improve the quality of structural proteins of the dermis.[11] All these methods of collagen synthesis activation can be grouped into five main blocks[12]: (1) Controlled injury: Skin damage by chemical (various peels), physical (lasers), or mechanical (dermarollers) factors, provided that the extent, severity, and depth of damage are controlled, triggers subsequent repair with collagen synthesis activation; (2) Photo- and thermal stimulation: These methods differ from the previous methods, in that they do not cause tissue destruction, i.e., they are gentler. These include non-ablative lasers and radiofrequency technologies, high-intensity focused ultrasound, and intense pulsed light[13]; (3) Biological stimulation: This is an opportunity to activate the synthetic function of fibroblasts by introducing various signaling molecules into the body.[14] Such molecules can be anabolic hormones, retinol, collagen, matrikins, growth factors, peptides, and components of placental products. The administration methods can vary from oral administration as part of dietary supplements and medicinal products to dermal application and injections.[14]; (4) Mechanical stimulation: The change in the tension of fibroblast membranes is also a vital factor in their synthetic function stimulation. Therefore, the introduction of bulk fillers and filaments leads to the activation of perifocally located fibroblasts and the deposition of collagen around the implant[14]; and (5) Chemical stimulation: A range of chemical compounds have a pronounced stimulating effect on fibroblasts, and the duration of the effect directly depends on the rate of product degradation.[12] This is how the PLA, calcium hydroxyapatite, and PCL function. To a certain extent, the effect of low concentrations of organic acids can also be attributed to this type of stimulation, although the effect is weak and short.
3. PLA: a safe and effective neocollagenogenesis stimulant
PLA is a biodegradable, immunologically inert, hypoallergenic, biocompatible product that does not accumulate in tissues.[1] Biodegradation of PLA occurs because water molecules break ether bonds that form the basis of the polymer[1]; however, owing to its hydrophobic nature, degradation occurs slowly, taking 2–5 years. Degradation products (lactic acid and its short oligomers) are recognized and metabolized by the body itself. By-products of PLA degradation are broken down during normal metabolic processes without causing an inflammatory or allergic reaction.[15] Over time, the molecular weight of PLA in tissues decreases (during non-enzymatic hydrolysis involving water molecules), and its molecules break down into lactic acid monomers (the process takes 12–18 months), which, in turn, decompose to carbon dioxide and water during the Krebs cycle reactions.[16] Data from the studies conducted on animal models, during which PLA labeled with radioactive carbon 14C and implanted in the anterior abdominal wall of rats, showed that the medicinal product was distributed at the injection site and was absent in the lymph nodes and other organs throughout the entire period of biodegradation.[16] After administration of the PLA product, immediate visual restoration of volume occurs, although this is largely due to mechanical damage of tissues and accompanying edema.[17] Then, a minor inflammatory reaction develops in the surrounding tissues, and PLA particles stimulate fibroblast proliferation and collagen synthesis. Subsequently, mature type I collagen fibers gradually form, and the skin surface smoothens (Fig. 2).
4. Chirality and biological activity
In nature, PLA has three isomeric forms (Fig. 3). Lactic acid and PLA exhibit optical activity, i.e., they exist in the form of two L- and D-stereoisomers and represent mirror images of each other.[18] Chirality is the property of a molecule not to combine with its mirror image in space. This term is based on the ancient Greek name of the most recognizable chiral object. Thus, the left and right hands are mirror images of each other but cannot be combined in space. Multiple biologically active molecules possess chirality, and natural amino acids and sugars are represented in nature mainly as one specific enantiomer [19]: amino acids mostly have an L- configuration, and sugars have a D-configuration. The enantiomeric forms of a molecule usually exhibit different biological activities, possibly because receptors, enzymes, antibodies, and other elements in the body also possess chirality, and a structural mismatch between these elements and chiral molecules prevents their interaction.[19]
5. Stereochemistry and material properties
Currently, lactic acid is obtained by microbiological synthesis.[20] The effectiveness of its biosynthesis process depends mainly on the producing microorganism, the cost of a substrate, and cultivation modes.[21] Microorganisms can synthesize both stereoisomers simultaneously and each of them separately. Synthesis depends on the presence of relevant lactate dehydrogenases.[22] Although both enantiomers are used in industrial practice, the L-(+)-LA isomer is of interest for biomedical applications, as it is involved in the cellular metabolism of the human body and reduces the risk of adverse reactions.[23] In the in vivo conditions, L-(+)-LA can be either included in the Krebs cycle or converted into glycogen in the liver; eventually, it is excreted from the lungs in the form of water and carbon dioxide.[23] PLA can be obtained from pure isomers of L-lactic and D-lactic acids, leading to the formation of poly-L-lactic (PLLA) and poly-D-lactic (PDLA) acid homopolymers, respectively.[23] If a racemic mixture of L- and D-monomers is used, a copolymer of poly-D,L-lactic acid (PDLLA) is obtained. Stereochemistry has a significant effect on the material properties: PLLA is a semi-crystalline polymer, and PDLLA is an amorphous polymer without a melting point. Besides, the degradation rate of PLLA is significantly lower than that of PDLLA due to the presence of crystalline regions.[23]
6. Mechanism of collagen-stimulating action of PLA
The process of collagen production stimulation after PLA injection was studied in both animal models and humans. Results of preclinical studies in animal models were validated and confirmed by the results of subsequent studies in humans. Interestingly, the biological effects and tolerability of PLA vary greatly depending on the stereoisomer used. In a study by Gao et al.[24] in laboratory animals, intradermal PLLA induced a significantly lower inflammatory response than PDLA and PDLLA. The expression of inflammatory cytokines (interleukin [IL]-1β, IL-6, and tumor necrosis factor [TNF]-α) around the injection site induced by PDLA was 3.2, 2.5, and 2.0 times higher, respectively, than that induced by PLLA and 2.3, 1.9, and 1.4 times higher than that induced by PDLLA.[24] Moreover, PLLA significantly stimulated collagen synthesis, which was 1.4 and 1.1 times higher than that induced by PDLA and PDLLA, respectively. These results confirm the superiority of PLLA as a dermal filler.[24]
7. Preclinical and clinical perspectives
Both preclinical and clinical studies of tissue response to PLLA in humans illustrate the subclinical inflammatory response to a foreign body weakening over time, leading to encapsulation of microparticles with subsequent degradation of PLLA and deposition of type I collagen in the ECM (Fig. 4).[25] The body’s reaction to a foreign material involves three consecutive events: protein absorption, cell recruitment, and fibrous encapsulation.[26] Protein absorption occurs immediately after injection and within the next hours after implantation of a PLLA product, followed by infiltration of damaged tissues by neutrophils and then by macrophages.[27] Proteins absorbed on the biomaterial surface include albumin, complement fragments, fibrinogen, fibronectin, immunoglobulin G, and vitronectin.[28] All of them are chemoattractants that attract inflammatory cells.[29] The attraction of inflammatory cells is also mediated by histamine, which is released as a result of mast cell degranulation.[30] As a result, monocytes and helper Th2 cells penetrate the area of PLLA injection.[31] Macrophages formed from monocytes also release signaling molecules, attracting additional macrophages to the implant.[32]
8. Macrophages and fibroblasts: catalysts of collagen synthesis in PLA injection
In the next stage of cellular reaction to the injected PLLA, macrophages, which are found in large numbers in the cellular infiltrate in close proximity to PLLA particles, play a main role.[33] If a foreign material cannot be phagocytized, epithelioid cells and macrophages merge under the influence of IL-4 and/or IL-13, with the formation of foreign body giant cells (FBGC) and a fibrous capsule.[34] As a result of these processes, PLLA microparticles are encapsulated for 3 weeks, and by 1 month after injection, they become surrounded by mast cells, mononuclear macrophages, foreign body cells, and lymphocytes.[35] Macrophages are the key cells that activate fibroblasts for the proliferation and synthesis of collagen.[35] They produce profibrotic factors, such as transforming growth factor beta (TGF-β1) and platelet-derived growth factor (PDGF), which support the immigration of fibroblasts and stimulate local fibroblasts to produce ECM collagen, eventually leading to encapsulation of foreign material.[36] While TGF-β appears to be a key mediator of collagen synthesis and fibroblast differentiation into α-actin-rich smooth muscle myofibroblast, PDGF promotes myofibroblast proliferation.[36] The differentiation of fibroblasts into myofibroblasts is essential for the formation of granulation tissue.
9. Immunofluorescence analysis of PLLA-treated tissue
To confirm a reaction to PLLA, the cellular infiltrate of the PLLA-treated tissue was examined by immunofluorescence, enabling the detection of macrophages near the PLLA and fibroblasts at a distance.[32] Immunofluorescence staining of the PLLA-treated tissue revealed a significant deposition of type III collagen in the area adjacent to the PLLA within the granuloma, while myofibroblasts deposit fibrous type I collagen on the capsule periphery.[37] Interestingly, the mRNA expression of type I and type III collagen is significantly increased immediately after the first injection of PLLA.[37]
After 3 months, a decrease in the inflammatory response was confirmed by the decreased number of cells. At this point, an increase in the number of collagen fibers was also evident. After 6 months, the number of macrophages and fibrocytes continued to decrease, and the production of collagen continued to increase. At this 6-month mark, the inflammatory reaction stopped, i.e., the tissue condition returned to its baseline. However, a significant increase in the amount of type I collagen occurred along the periphery of PLLA encapsulation between the 8th and 24th months after injection and later, as collagenogenesis continued.[38] This finding proves that PLLA breaks up in human tissues much slower than that thought previously.
10. Influence of PLLA on collagen production and tissue reactions
In the aforementioned studies, the authors suggested that moderate inflammation induced by PLLA and the paracrine effect of FBGC were the principal mechanisms involved in increasing the production of new collagen within a few months of injection. Granulomatous reactions to PLLA-based fillers have already been described.[39] Histological studies have revealed reactions to foreign bodies and the formation of FBGC. However, neither the biological background of these reactions nor the resulting enhancing effect of PLLA injection has been studied to date, and the exact mechanisms by which PLLA can increase collagen production remain unknown. Clinical experience shows that the effect of facial soft tissue augmentation due to PLLA injection is noticeable already by 1 month after injection,[40], which is much faster than that reported previously. Thus, it can be assumed that PLLA can directly influence dermal fibroblasts.
11. PLLA enhances collagen synthesis in dermal fibroblasts
In 2019, Kim et al.[40] conducted research to evaluate the effect of PLLA on collagen synthesis and associated signaling pathways in cultured dermal fibroblasts. The authors cultured the Hs68 cell line (human dermal fibroblast) and stimulated cells with PLLA.[40] To this end, sterile water was added to dry PLLA powder (Sculptra®; Sanofi Aventis, France), resulting in a 0.1% concentration of PLLA in the culture medium. The expression of type I collagen mRNA was drastically increased, as revealed by real-time RT-PCR reaction during 48-h incubation. This indicated that PLLA increased the transcription of type I collagen mRNA in a much shorter period than that thought previously. To determine whether an increase in collagen mRNA transcription was accompanied by increased collagen protein synthesis,[40] the concentration of procollagen in the medium was measured in accordance with the manufacturer’s protocol (Takara Bio, Otsu, Japan).[41] The amount of procollagen under the action of PLLA was significantly increased, which fully corresponded to the increase in the transcription of type I collagen mRNA. Wrinkles are formed due to changes in the arrangement and structure of the dermis. Human wrinkle fibroblasts (WF) have a slightly different functional profile than the smooth skin fibroblasts (SSF) of a middle-aged person.[42] Normal, functionally active fibroblasts can reorganize collagen fibers, but with age, their migration abilities, as well as the ability to synthesize collagen, decrease. During aging, the mitochondrial function of fibroblasts is impaired, which is reflected by an increase in lactate levels, a decrease in their proliferation potential, and a decrease in contractility.[42]
12. PLLA as a collagen production stimulator
Courderot-Masuyer et al.[43] studied the ability of PLLA to compensate for metabolic activity decrease and restore the migration function of WF, and inhibit lactate synthesis in SSF. They used two different skin samples taken during skin-tightening surgery in three women (one from the wrinkled area on the face and the other from the smooth skin area). The authors found that PLLA introduction into culture increases the synthesis of type I collagen, restores the ability of fibroblasts to migrate, reduces the production of lactate in SSF, stimulates their proliferation, and improves migration.[43] These results indicate that PLLA acts as a collagen production stimulator in the skin and is suitable for wrinkle correction.[44]
13. Effectiveness of Gana Fill fillers
The Gana Fill family (Gana V; GCS Co., Seoul, South Korea) of collagen-stimulating products based on PLLA consists of two certified products manufactured in South Korea: Gana X and Gana V (Fig. 5a).[45, 46] These products contain PLLA, carboxymethylcellulose, and mannitol. The diameter of Gana Fill particles is 30–50 μm (Fig. 5b), which is comparable to the size of fibroblasts, which ranges from 20 to 25 μm in undifferentiated forms and up to 40–45 μm in mature forms. Particles of the Gana Fill implant do not have the acute angle characteristics of Sculptra (Galderma Pharma, Lausanne, Switzerland), which reduces the risk of uncontrolled inflammation and granulomas. The probability of granuloma development increases with an increase in the ratio between the surface area and the volume of filler particles and decreases due to their shape with sharp edges.[47] Gana Fill particles have a very porous surface with a pore diameter of 0.45–1.44 μm, which ensures better integration in tissues and fixation of fibroblasts. The porous structure of Gana Fill particles promotes stronger fixation and activation of macrophages and FBGC recruiting fibroblasts.[48] Notably, smooth spherical particles of most fillers cause less activation of macrophages than particles with a more rough surface.[49] Moreover, the high porosity of Gana Fill microparticles facilitates the dilution process.
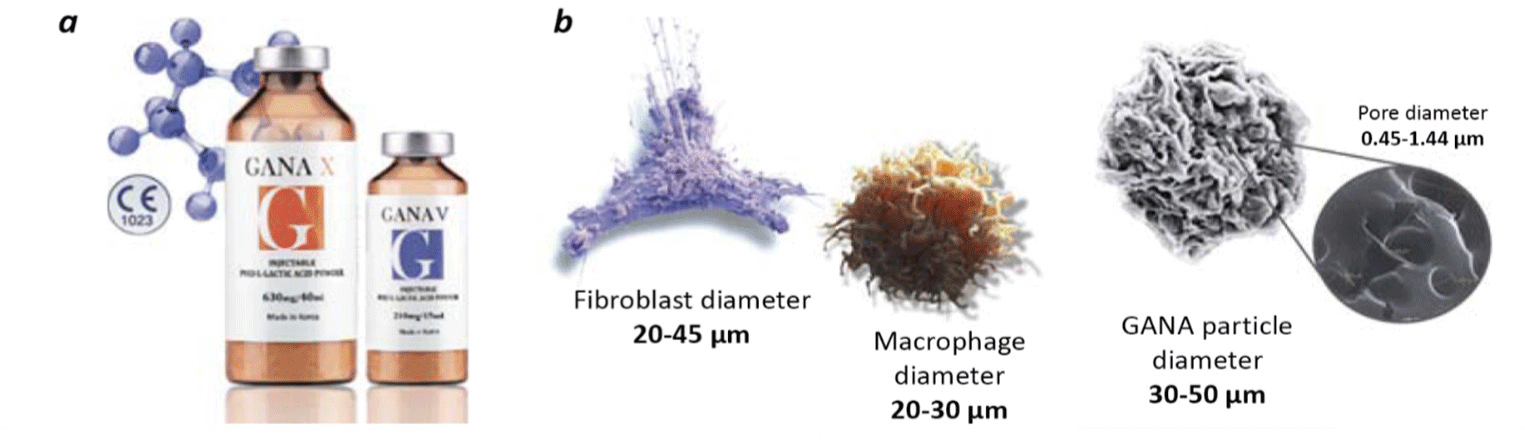
Table 1 presents the comparative characteristics of various PLA products registered in the Russian Federation, demonstrating a significant advantage of the Gana Fill in terms of parameters such as degree of crystallization, volume of PLLA in one vial, size of microgranules, and molecular weight.
14. Gana Fill fillers’ treatment procedure and prediction
The severity and onset rate of the effects depend on individual differences in age, type of aging, and skin quality.[50] PLLA injections are characterized by a gradually increasing effect; therefore, it is important to allow sufficient time for the main biological response to manifest before considering repeat procedures only when the outcome of the previous procedure becomes obvious.[51] This approach was previously described in the literature as the “treatment–expectation–evaluation” principle. At least 4–6 weeks should pass between the procedures.[52]
Although the increase in volume in the injection area may be noticeable immediately after injection, it occurs due to mechanical stretching of microparticles through suspension and disappears within a few hours or days. Nevertheless, it can provide an approximate idea of how the patient would look after 2 or 3 procedures, which enables the prediction of the number of procedures required to achieve the desired result.
When using PLLA, better clinical outcomes can be achieved due to a larger number of procedures performed, not because of an increased volume of the injected medicinal product, as is the case with the injection of hyaluronic acid-based fillers. However, to prevent hypercorrection and late adverse events, intervals between Gana Fill injection procedures should be observed, which should amount to 1–1.5 months. The results shall be evaluated no earlier than 6 months after the third procedure. This time interval is sufficient to determine the degree of correction achieved during the first three sessions and assess the need for additional procedures. This approach also minimizes the risk of hypercorrection of the injection area and reduces the risk of adverse events, including the development of papules and nodules.
15. Conclusion
Gana Fill fillers provide many opportunities for correcting the volumes and contours of soft tissues. The large molecular weight and high concentration of PLLA ensure a long-lasting pronounced effect. The highly porous structure allows for rapid preparation of the injectable product (approximately 10 min), and the optimal size and shape of the particles contribute to a significant reduction in complications.